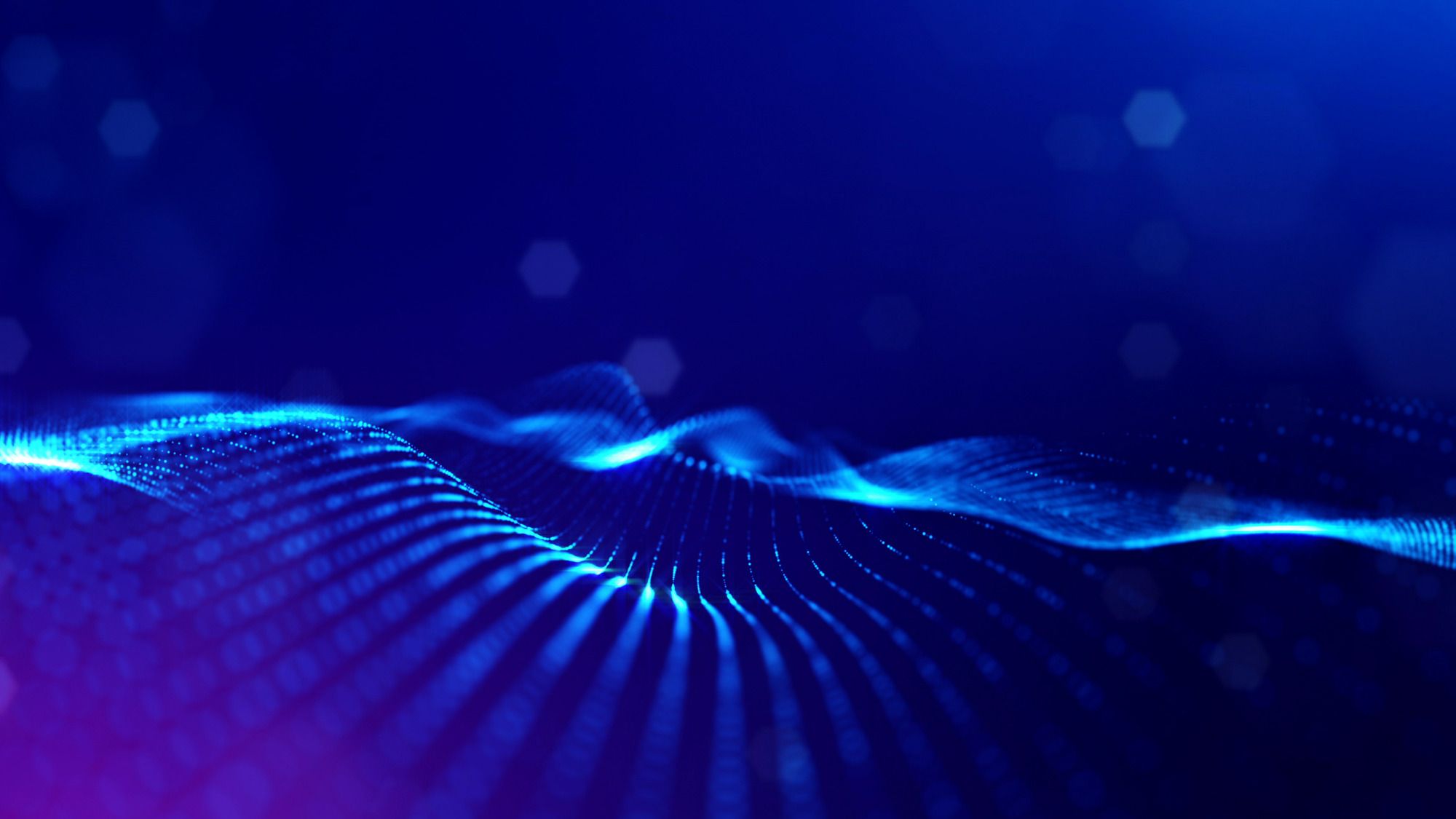
Past Voucher Award Recipient
Framatome Inc.
partnered with
Argonne National Laboratory
Modeling of Two-Phase Boiling Flow and Critical Heat Flux
with the Star-CCM+® and NEK-2P CFD Codes, NE-22-27436
YEAR AWARDED: 2022
TOTAL PROJECT VALUE: $684,248k
STATUS: Completed
PRINCIPAL LAB INVESTIGATORS: Adrian Tentner (ANL), Anca Hatman (Framatome
DESCRIPTION: Framatome is an international company that designs, manufactures, and installs components, fuel, and instrumentation & control systems for nuclear plants. Its mission is to be the world’s leading designer and supplier of nuclear steam supply systems, equipment, services, and fuel. Maintaining safe margins to critical heat flux (CHF) at nuclear plants is a central requirement for the Nuclear Regulatory Commission (NRC) in determining maximum operating power. At present, there is no computational fluid dynamics (CFD) commercialized technology available for CHF analyses that can predict the critical parameters for the onset of flow instability. This GAIN voucher project focused on modeling two-phase boiling flow and CHF prediction in realistic fuel-bundle geometries including specific spacer geometries.
BENEFIT: Argonne National Laboratory’s (ANL) previous experience in the development and implementation of the Extended Boiling Framework (EBF) in the CFD code STAR-CD and the Framatome experience and experimental data related to CHF occurrence provide the necessary collaborative environment to further the CHF predictive capability that will allow for the accelerated delivery of advanced fuel designs for light water reactors and potentially for advanced reactor fuel markets.
IMPACT: The extended Eulerian Multi-Phase (EMP) models developed during the project were implemented in the STAR-CCM+ CFD code and were first validated through simulations of multiple Becker benchmark CHF heated-pipe experiments performed under both dry-out (DO) and departure from nucleate boiling (DNB) conditions. The two-phase multi-flow regime model parameters and the constitutive correlations developed for the turbulence dispersion coefficient and vapor wall contact area fraction are valid for a wide range of mass, heat flux and system pressures. Good agreement between the experimental data and simulation results was observed for wall temperatures under boiling water reactor (BWR) DO conditions. For DNB conditions, the peak-wall temperatures were reasonably predicted with the modified wall-vapor heat flux model, but some deviations were found for post-DNB wall temperatures.
SIGNIFICANT CONCLUSIONS: Four fuel assembly benchmark tests were analyzed, and the DNB locations predicted by simulations were compared with the available experimental data from CHF experiments performed at Columbia University. The simulations predicted DNB locations that are in reasonable agreement with experimental data.
NEXT STEPS: Future work is recommended to enhance the extended STAR-CCM+ Eulerian Multiphase (EMP) models to further improve the post departure from nucleate boiling (DNB) wall temperature predictions in high pressure cases relevant for pressurized water reactor (PWR) conditions (165 bar and 200 bar). Also suggested are further analyses and model improvement for transition conditions (P=120 bar) between BWR and PWR conditions.